Cardiocurative effects of aqueous leaf extracts of Ximenia americana (linn.) and Pappea capensis (eckl. and zeyh.) against myocardial infarction in rats
Abstract
Different plants are traditionally applied in the treatment of different diseases, including chest pains, hypertension, and inflammation, among others. This study scientifically evaluated the curative treatment effects of X. americana and P. capensis leaf aqueous extracts in rats induced with myocardial infarction. The rats were randomly distributed as follows: Normal control group, consisting of rats treated with oral normal saline; Negative control group, comprising rats induced with MI and treated with normal saline; Positive control group, consisting of rats induced with MI and orally treated with propranolol (10 mg/Kg bw); and three experimental groups consisting of rats induced with MI and treated with plant extracts at dose levels 150, 200 and 250 mg/Kg bw. This study’s findings demonstrated that there was a significant reduction in the amounts of cTnT, LDH-1, CK-MB, T-cholesterol, triglyceride, LDL-c, and malondialdehyde, as well as a significant increase in levels of HDL-c, catalase, SOD, and glutathione peroxidase among rats treated with propranolol and plant extracts as compared to the negative control rats. Additionally, LC-MS analysis revealed the presence of flavonoids, tannins, and phenolics, which could explain the observed cardiocurative effects. This study concluded that aqueous leaf extracts of X. americana and P. capensis possess cardiocurative activities in rats with myocardial infarction. However, further studies on isolated fractions of the phytochemicals present are recommended. This study provides preliminary data useful in the development of pure and safe cardioactive compounds.
INTRODUCTION
Globally, cardiovascular diseases (CVDs) are the leading cause of mortalities and were responsible for approximately 16.7 million CVD-related global deaths in 2010, with predictions showing that this number will raise to about 23.3 million deaths by 2030 [1]. Moreover, data from the world health organization (WHO) show that CVDs caused approximately 17.9 million in 2019, which represents 32% of total global deaths [1]. Some of the well-known CVDs include myocardial infarction (MI), coronary artery disease (CAD), and angina pectoris, with MI being responsible for most CVD-related deaths [2]. Myocardial infarction refers to necrosis of the myocardium following reduced oxygen levels caused by impaired coronary blood flow [3]. Smoking, hyperlipoproteinemia, hypercholesterolemia, diabetes, aging, hypertension, and obesity are the major risk factors for MI [4].
Prescription and administration of cardiovascular drugs help reduce mortality and morbidity associated with myocardial infarction (MI) [5]. The treatment involves the administration of aspirin together with nitroglycerin and opioid analgesics, for chest pain management and supplemental oxygen in people with shortness of breath or low oxygen levels [6]. Moreover, thrombolytic drugs, percutaneous coronary intervention (PCI), and blood thinners, such as heparin, are recommended in cases with blockage [7]. However, the synthetic cardioactive drugs used in the treatment of MI are reported to cause many side effects and arguably, are not affordable [8]. Therefore, herbal remedies continue to offer an attractive alternative because they are known to be arguably affordable and readily available [9].
Some of the herbal plants with activity against cardiovascular diseases include Chung Hun Wha Dam Tang (CHWDT), a traditional Korean herbal formula with inhibitory effects on atherosclerosis-induced oxidative stress and inflammation via the NF-κB pathway [10]; Croton zambesicus that contains cardiac glycosides and phenols, which are active against MI [11]; Salvia miltiorrhiza (Danshen), a traditional Chinese herbal medicine, commonly used for the prevention and treatment of CVDs [12]; Mangifera indica containing cardioprotective flavonoids [13]; and Trichopus zeylanicus that contains cardioactive glycosides, tannins and flavannoids [14].
Many communities in Africa, including Mbeere, Aembu, and Ameru in Kenya, use extracts of X. americana and P. capensis in the treatment of different vascular and other heart-related ailments [15]. The published evidence shows that the two plants possess excellent antioxidant activities which could justify their application in the treatment of various pathologies [16; 17]. Nevertheless, the use of the two plants in the traditional management of vascular and heart-related diseases is not supported by sufficient scientific evidence, hence the need to scientifically evaluate their effectiveness. Therefore, this study was designed to scientifically evaluate the in vivo cardiocurative treatment effects of X. americana and P. capensis leaf aqueous extracts in rat models induced with MI. The current study confirmed the efficacy of the two extracts. The data reported in this study could be useful in the synthesis of pure, safe, and efficacious drugs for the treatment of MI.
MATERIALS AND METHODS
Chemicals
The reagents utilized in liquid chromatography-mass spectrometry (LC-MS) analysis included: methanol of high-performance liquid chromatography (HPLC) grade, acetonitrile (VWR UK), Milli-Q system purified water (Millipore Bedford MA USA), 98-100% formic acid (Tedia Inc USA), and Rutin reference standard (Sigma Aldrich USA).
Plant material collection and extraction
With assistance from local herbalists, fresh leaves of X. americana and P. capensis were collected from the Nthawa Ward in Mbeere-North Sub-County, Embu County, Kenya. A certified taxonomist carried out the plant samples’ botanical identification, and voucher specimens (voucher numbers DMG001 and DMG002 for X. americana and P. capensis, respectively) were deposited in the National Museums of Kenya’s Herbarium in Nairobi for future use. In the absence of direct sunlight, the freshly harvested plant leaves were thoroughly dried at room temperature.
An electric mill was used to grind dried leaves into a fine powder. Separately, 250 grams of each powdered plant material were soaked in 2 liters of distilled water in a conical flask, put in a water bath, and left to stand for 2 hours at a temperature of 60 degrees Celsius. Decanting was then done, and using filter paper (Whatman No.1), the resulting solution was filtered and filtrate dried in a freeze drier [18]. The resultant dry extracts from the two plants were separately packaged and stored in airtight containers in a refrigerator at -4°C before they were used to conduct the experiments [19].
Experimental animals
Wistar albino rats (Rattus norvegicus), either gender, aged from 10 to 11 weeks and weighing 250±10 grams were used for bioassays. The breeding and experimentation unit at the department of Biochemistry, Microbiology, and Biotechnology, Kenyatta University, was used for animal handling and breeding. Standard propylene cages were used to house the animals and standard laboratory conditions such as room temperature (250C) and twelve hours of light succeeded by twelve hours dark cycles, which were maintained throughout the experiments. The rats were supplied with water ad libitum and fed on standard rodent pellets. The study adhered to ethical considerations and protocols for maintaining and handling experimental subjects [20, 21]. Institutional Animal Ethics Committee, Kenyatta University, as well as National Commission for Science, Technology, and Innovation (NACOSTI) reviewed and approved the protocols used in the experiments (Approval numbers PKUA/006/006 and NACOSTI/P/21/8549, respectively).
Induction of MI
Myocardial infarction was induced with salbutamol at a dose level of 7.5 mg/Kg bw that was administered intraperitoneally once a day for 2 consecutive days, following which blood samples were taken to check levels of cardiac function biomarkers [22].
Experimental design
Animals were randomized into six sets each comprising five animals. In group I, the normal control, MI was not induced, and rats were only given oral 0.9% w/v normal saline. Group II (Negative control) was made up of salbutamol-stimulated MI rats that received 0.9% w/v normal saline only. Group III (Positive control group) comprised MI rats treated with oral 10 mg/Kg bw propranolol. In groups IV, V and VI, MI was induced, and rats were treated with oral extract dose of 150, 200 and 250 mg Kg-1 bw-1, correspondingly (Table 1).
Animals were induced with MI on days 1 and 2 and then treated daily for 14 days (until day 16). On the 17th day, body weights of the animals were taken, euthanization done and each animal’s heart carefully removed and weighed. On days 1 and 2, blood samples for biochemical analyses were taken from retro-orbital plexus, whereas on day 17, blood was taken by cardiac puncture.
Before drawing blood on days 1 and 2, anaesthetization was done using intraperitoneal ketamine (75 mg/Kg) and xylazine (8.0 mg/Kg). Euthanization was done using overdose isoflurane in a vacuum glass desiccator. Well-labelled vacutainer tubes were utilized for the collection of blood samples, and centrifugation was done to collect serum for biochemical analysis. The serum was used for the determination of cardiac function biomarkers and lipid profiles. Cardiac antioxidant biomarkers were determined using freshly harvested heart tissues.
Table 1. Treatment protocol for the evaluation of curative treatment effects of aqueous leaf extracts of X. americana and P. capensis.
Determination of relative heart weight
The animals’ body weights were recorded on the day of euthanization. Heart weight was measured after carefully harvesting the heart, squeezing out blood, blotting on the filter paper, and washing in ice-cold saline. A digital weighing balance (Mettler PJ 3000) was used. Relative heart weight was computed using the formula given by Lazic et al [23].
Determination of cardiac function biomarkers
Cardiac function biomarkers, including creatine kinase-MB (CK-MB) fraction, lactate dehydrogenase-1 (LDH-1), and cardiac troponin T (cTnT) were determined. Cobas E 411 -Roche machine was used to determine serum cardiac troponin T using Roche cassettes, whereas lactate dehydrogenase-1 and creatine kinase-MB fraction were determined using Cobas Integra 400 Plus from Roche.
Determination of lipid profiles
The lipid profiles including total cholesterol (T-Chol), triglycerides (TG), and high and low-density lipoprotein-cholesterol (HDL-c and LDL-c) were analyzed using a chemistry autoanalyzer (Mindray BS 120).
Determination of antioxidant enzymes
After animals were sacrificed, their hearts were carefully removed, homogenized, and then centrifuged to obtain supernatant, which was used in the determination of cardiac antioxidant enzyme levels. The enzymes included superoxide dismutase (SOD), catalase (CAT), and glutathione peroxidase (GPx) [24; 25]. Malondialdehyde (MDA) levels were also measured as described [26].
Liquid chromatography-mass spectrometry (LC-MS) analysis
A model 1200 quaternary LC pump, connected to a single quadruple MS with electrospray source, Agilent MSD 6120 -Palo Alto, CA, and Hewlett-Packard chemStation control system software, were the features and operating conditions for LC-MS. Reverse-phase liquid chromatography was done using an Agilent technology 1200 infinite series (Zorbax SB) stationary phase, with a C18 column of 1.8 micrometers by 2.1 and 50 millimeters (Phenomenex, Torrance, CA). The mobile state included water and acetonitrile with a gradient program of 0 minute, 5 percent B; 0 to 5 minutes, 5 to 50 percent B; 5 to 10 minutes, 50 to 80 percent B; 10 to 15 minutes, 80 to 100 percent B; 15 to 25 minutes, 100 percent B; 25 to 30 minutes, 100 percent B; 30 to 35 minutes. The flow rate was constantly held at one milliliter per minute, whereas the injection volume was at 20 microliters.
Data were collected utilizing a 100 -1500 m/z scan range in full-scan positive-ion mode. Each ion’s residence duration was 50 milliseconds, and the mass spectrometer’s other specifications were as follows: 3 kilovolts capillary voltage, 70 volts cone voltage, 5 volts extract voltage, 0.5 volts RF voltage, 110 degrees Celsius source temperature, 380 degrees Celsius nitrogen gas desolvation temperature, and 400 liters per hour nitrogen gas desolvation flow. Phytocompounds were identified based on their general fragmentation patterns and reference spectra in MS databases of the National Institute of Standards and Technology.
Data processing and statistical analysis
Data on relative heart weight, cTnT, CK-MB, LDH-1, T-cholesterol, triglyceride, LDL-c, HDL-c, GPx, CAT, SOD, and MDA amounts were recorded in the spreadsheet (Microsoft® Excel), organized, then transferred to statistical software (Minitab v19, NC, USA) for analysis. It was subjected to the D’Agostino-Pearson test and confirmed to satisfy the basic assumptions of parametric data. Descriptive statistics were done, and results were presented as Mean ± standard error of Mean. One-factor ANOVA was utilized for inferential statistical analysis. Tukey’s post hoc was done for pairwise mean comparisons and separation. The statistically significant differences were inferred at a p-value of ≤ 0.05, whereas the results were presented in tables and visualized in graphs
RESULTS
Effects of aqueous leaf extracts of X. americana and P. capensis on relative heart weight in myocardial infarction-induced rats
Generally, the current study showed that the aqueous leaf extracts of X. americana and P. capensis significantly reduced (p < 0.05) relative heart weight in MI rats compared with the weights in the negative control rats (Table 2). The results established that the effects of the extracts were dose-dependent (Table 2). It was also noted that X. americana extract at 250 mg Kg-1 bw-1 and propranolol reduced the relative heart weight of MI rats to normal (Table 2). However, at 150 and 200 mg Kg-1 bw-1, the extract did not reduce the relative heart weight to normal (Table 2). In addition, at 200 and 250 mg Kg-1 bw-1 the extract was as effective as the reference drug, propranolol (Table 2). Notably, the difference between the effects caused by X. americana extract at 150 and 200 mg Kg-1 bw-1 were significant (p ˃ 0.05; Table 2). Similarly, P. capensis extract, at 250 mg/Kg bw dose level, reduced relative heart weight of rats to normal (Table 2). However, 150 and 200 mg Kg-1 bw-1 dose levels of P. capensis extract did not reduce relative heart weight to normal (Table 2). Additionally, at 150 and 200 mg Kg-1 bw-1, the extract showed significantly similar activity (p ˃ 0.05; Table 2). Moreover, P. capensis doses of 200 and 250 mg Kg-1 bw-1 showed comparable (p ˃ 0.05) activity to that exerted by propranolol (Table 2). In comparison, the current study established that the cardioprotective effects of the two plant extracts were comparable at the corresponding doses (p ˃ 0.05; Figure 1).
Table 2. Effects of aqueous leaf extract of X. americana and P. capensis on relative heart weight in rats induced with myocardial infarction.
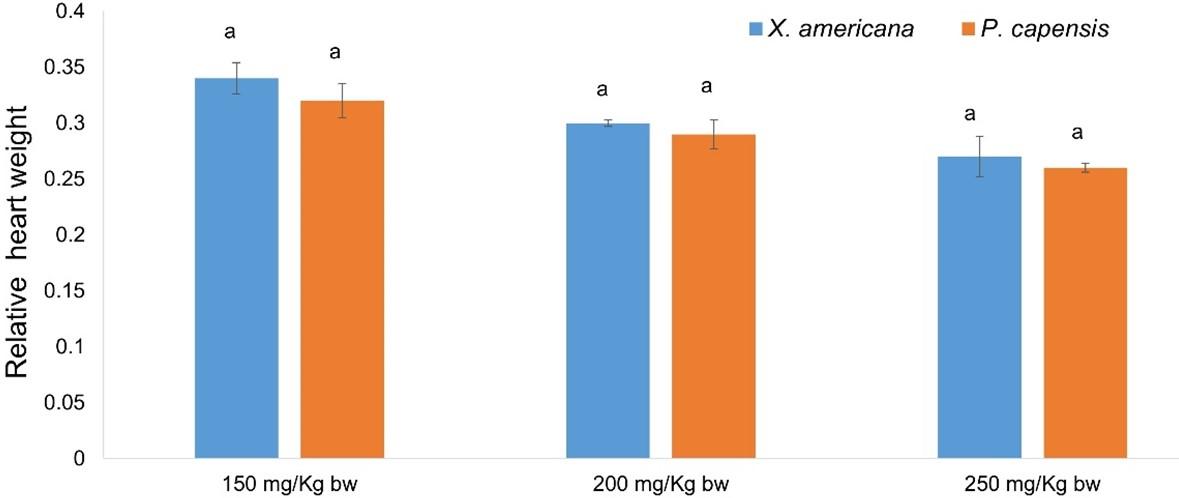
Effects of aqueous leaf extracts of X. americana and P. capensis on serum cardiac troponin T levels in myocardial infarction-induced rats
Overall, the current study established that X. americana and P. capensis extracts significantly reduced serum cTnT levels in extract-administered rats as compared to negative control rats (p < 0.05; Table 3). In general, the effects of the extracts were dose-dependent (Table 3). For X. americana extract-treated rats, the extract dosage of 250 mg/Kg bw and propranolol reduced cTnT levels to normal (Table 3). Moreover, the extract at 200 and 250 mg Kg-1 bw-1 was as effective as propranolol (p ˃ 0.05; Table 3). Additionally, there was no significant difference (p ˃ 0.05) between the effects caused on cTnT levels by 200 and 250 mg Kg-1 bw-1 dose levels of X. americana extract (Table 3). Further, the results showed that P. capensis extract dosages of 200 and 250 mg Kg-1 bw-1 effectively decreased serum cTnT to normal levels (Table 3). However, 150 mg/Kg bw dose of P. capensis extract did not effectively reduce serum cTnT levels to normal (Table 3). Moreover, there was no significant difference (p ˃ 0.05) in the effects caused by the extract at 200 and 250 mg Kg-1 bw-1 as compared to propranolol (Table 3). Generally, P. capensis extract showed significantly better (p < 0.05) serum cTnT reduction compared to X. americana extract at the three corresponding extract doses (Figure 2).
Table 3. Effects of aqueous leaf extract of X. americana and P. capensis on serum cTnT levels in rats induced with myocardial infarction.
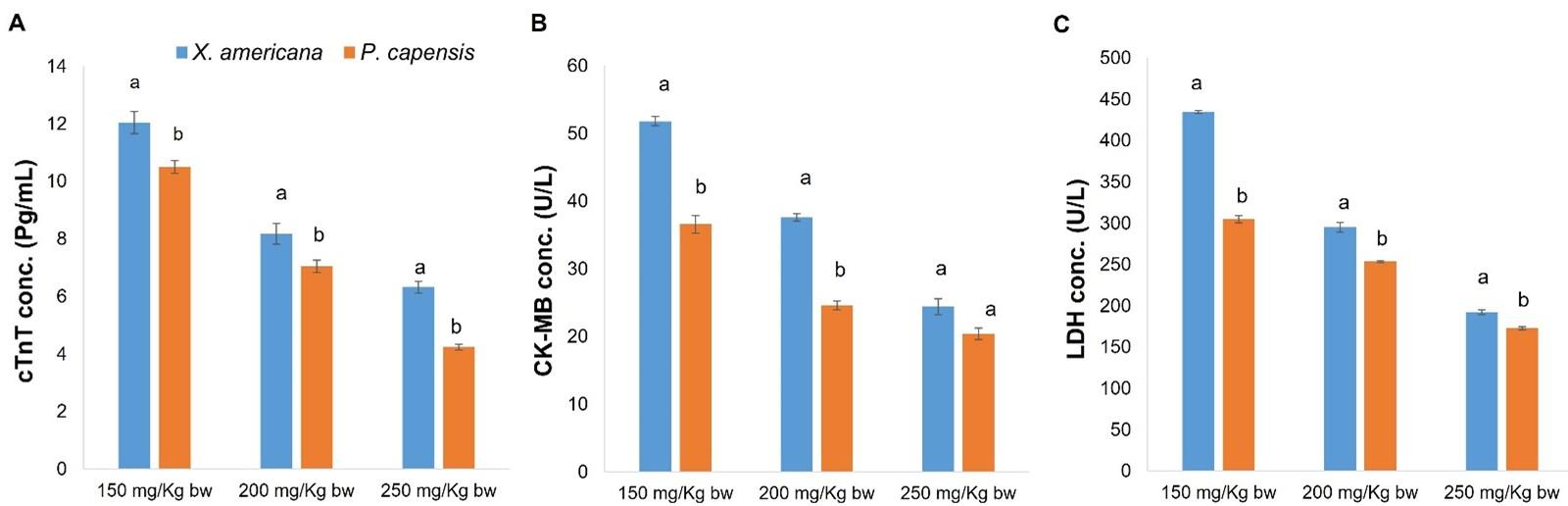
Effects of aqueous leaf extracts of X. americana and P. capensis on creatine kinase-MB (CK-MB) levels in myocardial infarction-induced rats
As table 4 show, X. americana and P. capensis extracts significantly reduced (p < 0.05) serum CK-MB concentration of MI rats as compared to negative control rats. Generally, the study findings showed that the extracts reduced CK-MB levels in a dose-driven trend (Table 4). For the X. americana extract-treated MI rats, 150 and 200 mg Kg-1 bw-1 dose levels of the extract did not reduce serum CK-MB levels to normal (Table 4). However, the extract dose of 250 mg Kg-1 bw-1 decreased serum CK-MB to normal levels (Table 4). In addition, X. americana extract at dosage of 250 milligrams/Kg bw and propranolol exerted comparable (p ˃ 0.05) effects on levels of serum CK-MB (Table 4). On the other hand, P. capensis extract at 150 mg Kg-1 bw-1 dose level did not reduce CK-MB levels to normal levels (Table 4). However, 200 and 250 mg Kg-1 bw-1 dose levels of the extract reduced serum CK-MB levels to normal levels (Table 4). Additionally, in reduction of CK-MB levels, the extract at 200 and 250 mg/Kg bw dose levels was as effective as propranolol (p ˃ 0.05; Table 4). Further, there was no significant difference (p ˃ 0.05) between levels of serum CK-MB in rats treated with 200 and 250 mg Kg-1 bw-1 dose levels of P. capensis extract (Table 4). In comparison, P. capensis extract at 150 and 200 mg Kg-1 bw-1, showed significantly greater reduction of serum CK-MB levels as compared to the corresponding X. americana extract doses (p < 0.05; Figure 2). However, at 250 mg Kg-1 bw-1 dosage, the effects of the two extracts on serum CK-MB levels were statistically similar (p ˃ 0.05; Figure 2).
Table 4. Effects of aqueous leaf extract of X. americana and P. capensis on serum CK-MB levels in rats induced with myocardial infarction.
Effects of aqueous leaf extracts of X. americana and P. capensis on serum lactate dehydrogenase-1 levels (LDH-1) in myocardial infarction-induced rats
Generally, X. americana and P. capensis extracts significantly decreased (p < 0.05) serum LDH-1 concentration in extracts-treated MI rats as compared with the amount in negative control rats (Table 5). The activity of the two plant extracts on LDH-1 were dose-dependent (Table 5). It was noted that X. americana extract at 250 mg Kg-1 bw-1 dose level and propranolol reduced LDH-1 levels to normal (Table 5). However, at dosages of 150 and 200 mg Kg-1 bw-1, the extract did not reduce LDH-1 levels to normal (Table 5). In addition, the findings showed that the effects of X. americana extract at 250 mg Kg-1 bw-1 and propranolol were comparable (p ˃ 0.05; Table 5). However, 150 and 200 mg Kg-1 bw-1 dose levels of the extract exhibited significantly lesser (p < 0.05) effects compared to propranolol (Table 5). Similarly, at 250 milligrams Kg-1 bw-1 dose level, P. capensis extract reduced serum LDH-1 levels to normal (Table 5). However, at 150 and 200 mg/Kg bw dose levels, the extract did not reduce LDH-1 levels to normal (Table 5). Moreover, at dosages of 250 mg/Kg bw, the efficacy of P. capensis extract in reduction of LDH-1 levels was comparable (p ˃0.05) with that of propranolol (Table 5). However, the extract at 150 and 200 mg Kg-1 bw-1 dose level, caused significantly lesser (p < 0.05) reduction of LDH-1 levels as compared with that of propranolol (Table 5). In comparison, the three P. capensis extract dose levels caused significantly greater effect on LDH-1 levels as compared with the corresponding X. americana extract dose levels (p < 0.05; Figure 2).
Table 5. Effects of aqueous leaf extract of X. americana and P. capensis on serum LDH-1 levels in rats induced with myocardial infarction.
Effects of aqueous leaf extracts of X. americana and P. capensis on lipid profile levels in myocardial infarction-induced rats
In general, X. americana and P. capensis leaf aqueous extracts significantly decreased (p < 0.05) levels of LDL-c, triglycerides, and T-cholesterol in extracts-treated salbutamol-stimulated MI rats as compared to negative control rats (Table 6). On the other hand, the two extracts significantly raised (p < 0.05) levels of HDL-c in extracts-administered rats as compared with levels in negative control rats (Table 6). Overall, the effects of the two extracts were dose-dependent (Table 6).
For X. americana extracts-treated rats, 250 mg Kg-1 bw-1 dose level of the extract lowered LDL-c, total cholesterol, and triglyceride to normal levels (Table 6). In addition, X. americana extract at 250 mg Kg-1 bw-1 dose and propranolol exerted comparable (p ˃ 0.05) effects on HDL-c, LDL-c, cholesterol, and triglyceride levels (Table 6). Moreover, the X. americana extract, at 200 mg/Kg bw dose level, lowered triglyceride levels to normal (p ˃ 0.05; Table 6). Additionally, the effects caused by 200 mg/Kg bw dose level of X. americana extract on triglycerides were similar to those caused by propranolol as well as to those caused by the extract at 150 and 250 milligrams Kg-1 bw-1 dose levels (p ˃ 0.05; Table 6). Moreover, the current study revealed that at 150 milligrams Kg-1 bw-1, X. americana extract did not appreciably reduce levels of LDL-c. This is because LDL-c levels in rats administered with this extract dosage did not significantly differ (p ˃ 0.05) compared with those of negative control rats (Table 6). Similarly, 150 mg/Kg bw dose level of X. americana extract did not significantly increase (p ˃ 0.05) the levels HDL-c (Table 6).
On the other hand, 250 mg/Kg bw dose level of P. capensis extract increased HDL-c and decreased LDL-c, T-cholesterol and triglyceride to normal levels (p ˃ 0.05; Table 6). Moreover, the effects caused by 250 mg Kg-1 bw-1 dosage of the extract were similar to those caused by propranolol (p ˃ 0.05; Table 6). In addition, at 200 Kg-1 bw-1, the P. capensis extract decreased triglycerides and LDL-c normal levels (p ˃ 0.05; Table 6). In addition, it was showed that the effects caused on triglycerides and LDL-c by P. capensis extract at dose of 200 and 250 levels did not significantly vary (p ˃ 0.05; Table 6). Notably, 150 milligram Kg-1 bw-1 dose of P. capensis extract did not significantly reduce (p ˃ 0.05) LDL-c levels among extracted-treated rats as compared with the amount in negative control rats (Table 6). Moreover, the effects on triglycerides by P. capensis extract at 150 and 200 milligrams Kg-1 bw-1 were comparable (p ˃ 0.05; Table 6).
In comparison, 150 milligrams Kg-1 bw-1 dose level of P. capensis extract induced significantly greater (p < 0.05) decrease in T-cholesterol levels as compared with the levels exerted by the corresponding X. americana extract dose level (Figure 3). At 200 and 250 mg Kg-1 bw-1, the effects of the two extracts on levels of total cholesterol were statistically alike (p ˃ 0.05; Figure 3). Moreover, the two plant extracts showed comparable (p ˃ 0.05) effects, on triglycerides and LDL-c levels, at the three extract concentrations (Figure 3). However, 200 mg Kg-1 bw-1 dose of P. capensis extract caused significantly greater (p < 0.05) increase in HDL-c levels as compared to equivalent dose level of X. americana extract (Figure 3). It was further noted that at 150 and 250 milligrams Kg-1 bw-1, the effects on HDL-c by the two plant extracts were comparable (p ˃ 0.05; Figure 3).
Table 6. Effects of aqueous leaf extract of X. americana and P. capensis on lipid profile levels in rats induced with myocardial infarction.
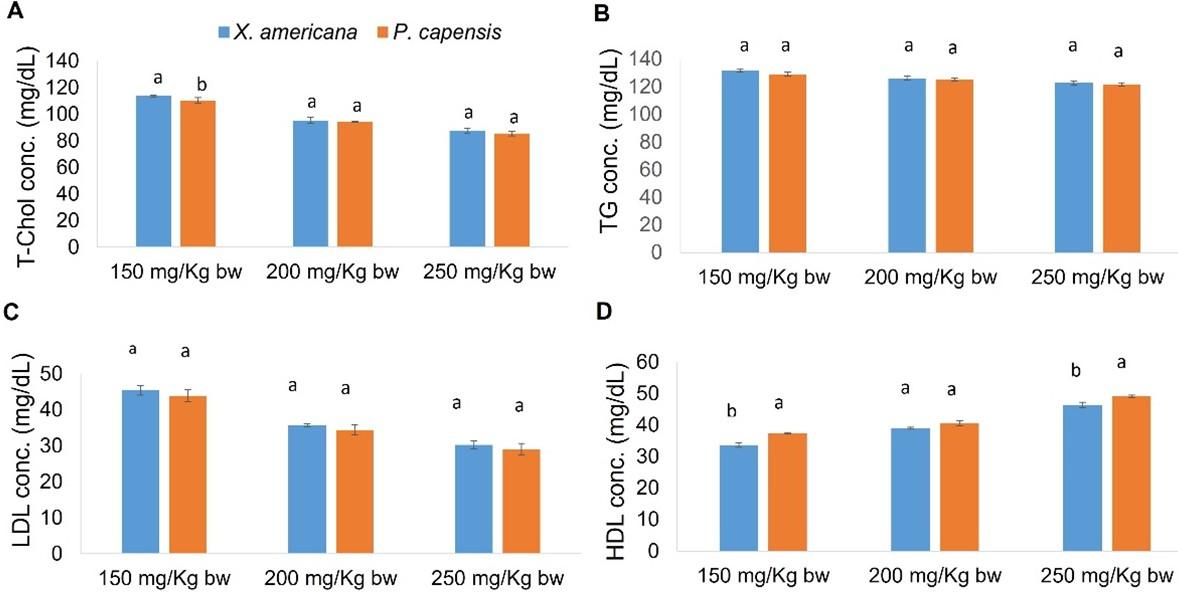
Effects of aqueous leaf extracts of X. americana and P. capensis on cardiac antioxidant enzymes levels in myocardial infarction-induced rats
Generally, the current study showed that the X. americana and P. capensis leaf aqueous extracts significantly reduced (p < 0.05) MDA levels and raised amounts of GPx, CAT and SOD in extracts-treated rats as compared with the concentration shown in negative control rats (Table 7). In general, the effects of the two extracts were dose-dependent (Table 7).
For X. americana extract-treated rats, the extract did not increase SOD, CAT and GPx levels to normal (Table 7). Moreover, the X. americana extract did not reduce MDA levels to normal (Table 7). Additionally, at dose level of 250 milligrams Kg-1 bw-1, the X. americana extract showed comparable (p ˃ 0.05) efficacy to propranolol (Table 7). Additionally, the extract, at 250 mg Kg-1 bw-1, caused significantly higher (p < 0.05) rise of GPx and CAT levels as compared with propranolol (Table 7). Notably, the effects caused on CAT levels by X. americana extract, at 200 milligrams Kg-1 bw-1, were not significantly different (p ˃ 0.05) as compared to those caused by propranolol (Table 7).
This study showed that 250 mg/Kg bw dose level of P. capensis extract increased CAT and reduced MDA levels to normal (Table 7). However, the P. capensis extract did not increase SOD and GPx levels to normal (Table 7). Generally, P. capensis extract at 250 mg/Kg bw caused significantly greater (p < 0.05) rise in GPx, SOD and CAT levels, as well as higher reduction in MDA levels as compared to propranolol (Table 7). In addition, the activity caused on CAT levels by 200 mg Kg-1 bw-1 dose level of P. capensis extract were comparable to levels caused by the propranolol (p ˃ 0.05; Table 7). However, at 150 and 200 milligrams Kg-1 bw-1 dose levels, P. capensis extract did not increase GPx, SOD and CAT levels to normal (p < 0.05; Table 7).
In comparison, the current study showed that P. capensis extract at 250 mg Kg-1 bw-1 caused significantly greater (p < 0.05) rise in SOD concentrations as compared to the corresponding X. americana extract dose level (Figure 4). Noteworth, 150 and 200 mg/Kg bw dose levels of the extracts exerted statistically similar (p ˃ 0.05) levels of SOD (Figure 4). Generally, the effects on CAT levels by the two plant extracts at the three corresponding doses were comparable (p ˃ 0.05; Figure 4). Conversely, the three P. capensis extract dose levels were significantly more effective, on GPx levels, as compared to the corresponding X. americana extract doses (p < 0.05; Figure 4). Moreover, this study showed that at 150 mg/Kg bw dose level, P. capensis extract caused significantly greater reduction of MDA levels as compared to the corresponding X. americana extract dose level (p < 0.05; Figure 4). Nevertheless, the two extracts at 200 and 250 milligrams Kg-1 bw-1 exerted comparable (p ˃ 0.05) reduction in MDA levels (Figure 4).
Table 7. Effects of aqueous leaf extract of X. americana and P. capensis on cardiac antioxidant enzymes levels in rats induced with myocardial infarction.
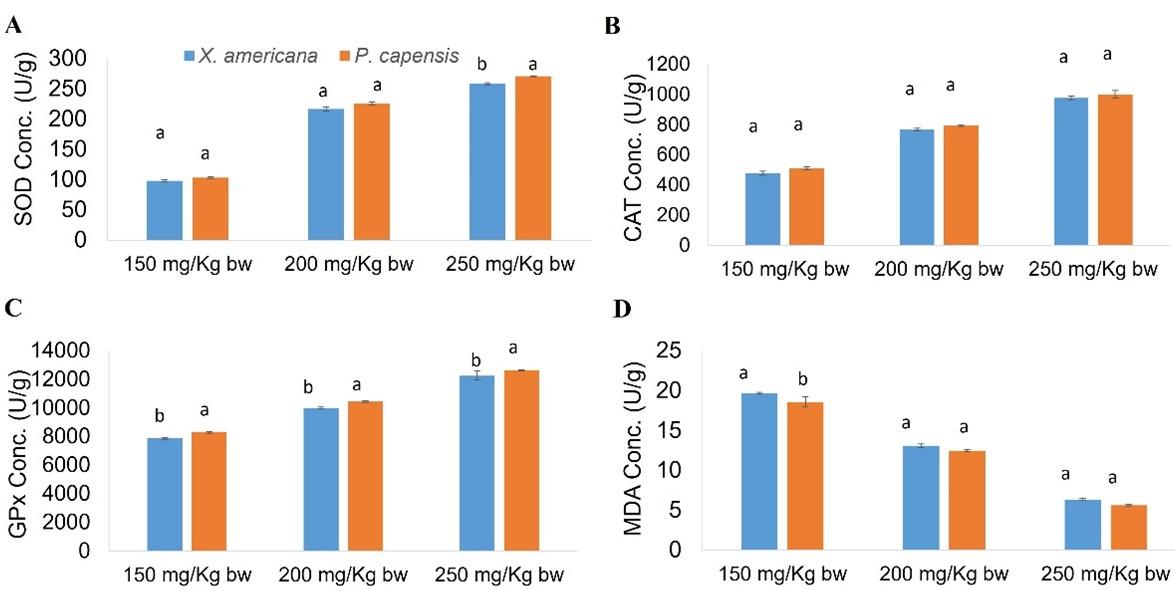
Phytochemical profile of aqueous leaf extracts of X. americana and P. capensis
The LC-MS analysis for X. americana showed presence of various phytochemicals, including flavonoids, tannins and phenolic acids (Table 8). On the other hand, LC-MS analysis for P. capensis showed presence of flavonoids, glycosides, and phenolic acids (Table 8).
Table 8. Identification and characterization of phytocompounds in leaf extract of X. americana and P. capensis.
DISCUSSION
Generally, the search for herbal medicines continue to gain interest because of limitations associated with conventional drugs, including side effects and high prices. Many herbal plants, such as X. americana and P. capensis, have been extensively researched on and proven to confer many therapeutic benefits. However, their traditional application in the treatment of MI has not been scientifically proven. Therefore, the scientific data on the effectiveness of these herbal plants in MI is crucial in validating the traditional application.
In this study, cardiocurative treatment effects of X. americana and P. capensis leaf aqueous extracts were determined in rats induced with MI. The first aspect for determining cardiocurative effects of the extracts was on relative heart weight of MI rats. The extracts’ effects on rats were compared to those recorded in normal and negative control rats. In this study, the findings showed that X. americana and P. capensis extracts significantly decreased relative heart weights of MI rats. The decrease was dose-depedent. These findings agreed with a past study done by Kang et al, which reported that Atractylodes macrocephala extracts caused dose-depedent decrease in heart weight index of rats induced with chronic heart failure [27]. Similarly, Ayza et al reported that stem bark extract of Croton macrostachyus showed notable relative heart weight of rats induced with cardiotoxicity [28]. The effects of the extracts on relative heart weight could be due to various secondary metabolites present, which prevented cardiac hypertrophy by alleviation of oxidative stress. Such phytochemicals include flavonoids that possess vasodilatory activities, that reduces peripheral resistance and improves coronary circulation [28]. In addition, protocatechuic acid, also present in the extracts, has been associated with anti-apoptotic and pro-survival activities of heart muscle [29].
In addition, the X. americana and P. capensis extracts’ cardiocurative effects were determined based on serum levels of CK-MB, LDH-1 and cTnT. Reduction in cTnT, CK-MB and LDH-1 levels by the extracts was significant. The findings agreed with a previous research done by Nayagam et al on the cardioprotective effects of Caesalpinia bonducella (Linn.) in rats with MI induced with doxorubicin [30]. The effects of the extracts were thought to be due to the presence of phytocompounds which limited damage of cardiomyocytes and therefore, preventing leaking of cTnT, CK-MB and LDH-1 into the blood. Such phytocompounds include catechins and quercetins [31]. Catechins modulate apoptosis by regulation of gene expression for anti- and pro-apoptosis [21]. In addition, catechins confer anti-inflammatory effects by regulating different isoforms of nitric oxide synthase [32]. On the other hand, quercetins reduce oxidative stress through blockage of Fenton reaction, scavenging on reactive oxygen species directly and xanthine oxidase and NADPH oxidase inhibition. Moreover, quercetins have inhibitory vasodilation effects on endothelin-1 receptors. These effects lead to higher stimulation of NO and large-conductance calcium-activated potassium channels activation [33]. Since P. capensis extract contained more quercetin derivatives, it generally exhibited greater activity as compared to the X. americana extract.
Moreover, lipid profiles were evaluated as another way of evaluating cardiocurative effects of the extracts. Serum lipid levels increases in response to degradation of cell membranes as a result of salbutamol-induced oxidative stress. The lipid profiles determined in this study include TC, TG, LDL-c and HDL-c. Notably, X. americana and P. capensis extracts caused a significant decrease in LDL-c, T-cholesterol and triglycerides levels, as well as rise in levels of HDL-c. The anti-lipidaemic effects of the extracts could be due to the presence of phytochemicasl with antioxidative effects [31].
Finally, the levels of SOD, CAT, GPx and MDA were determined as another way of evaluating cardiocurative effects of extracts. The extracts increased levels of SOD, CAT and GPx, and reduced levels of MDA. This could be due to the presence of different phytochemicals with free radical scavenging and antioxidant gene upregulation activity [34; 35]. The activity of phytochemicals on nuclear factor erythroid 2-related factor 2 leads to increased levels of various antioxidant enzymes, including CAT, SOD and GPx [36]. Generally, the cardiocurative effects of X. americana and P. capensis could be due to the presence of various phytocompounds. These phytocompounds are believed to possess ability to scavenge free radicals, upregulate antioxidant and anti-inflammatory gene expression and be able to block beta adrenergic receptors. This lead to stabilization of membranes of cardiomyocytes thereby reducing leakage of intracellular components including cTnT, CK-MB, LDH-1 and lipids into the blood.
CONCLUSION
Findings of the current study indicated that the aqueous leaf extracts of X. americana and P. capensis confer cardiocurative activities in rats induced with myocardial infarction. However, further studies are recommended to test and compare cardiocurative treatment effects of various phytochemicals identified in the studied extracts.
ACKNOWLEDGEMENTS
This research received financial supported from Chuka University (Internal Research Fund-2021). The authors are thankful to Shadrack Njagi, Daniel Gathuka, Ann Wanjira and Daniel Gitonga, for their help in this work.
AUTHOR CONTRIBUTIONS
Gaichu wrote the first manuscript and performed statistical analysis. Study design, data collection, review and editing of the manuscript were performed by Gaichu, Ngugi and Mathabe. All authors read and approved the final manuscript.
CONFLICTS OF INTEREST
There is no conflict of interest among the authors.
References
- [1]Islam S, Nobel FA, Sabrina S, Islam A, Islam MJ. Assessment and comparison of cardiovascular disease risk factors and biochemical parameters among men and women: A cross-sectional study. J Adv Biotechnol Exp Ther. 2023; 6(1): 25-34.
- [2]Dagenais GR, Leong DP, Rangarajan S, Lanas F, Lopez-Jaramillo P, Gupta R, et al. Variations in common diseases, hospital admissions, and deaths in middle-aged adults in 21 countries from five continents (PURE): a prospective cohort study. The Lancet. 2020;395(10226):785-94.
- [3]Al-Anbari AA, Alta’ee AH, Al-Saad SF. A study of arginase-1 activity and lipid profile in patients with myocardial infarction. J Adv Biotechnol Exp Ther. 2022; 5(3): 553-561.
- [4]Obaidullah MM, Chowdhury MR, Islam S, Barmon AK, Ara I, Hossain MN. Analysis of the risk of cardiovascular diseases among people with diabetes according to triglyceride level. J Adv Biotechnol Exp Ther. 2021; 4(2): 210-214.
- [5]Bonow RO, Mann DL, Zipes DP, Libby P. Braunwald’s heart disease: a textbook of cardiovascular medicine. 11th ed. Philadelphia: Elsevier Science; 2011.
- [6]Fabris E, Selvarajah A, Tavenier A, Hermanides R, Kedhi E, Sinagra G, van’t Hof A. Complementary Pharmacotherapy for STEMI Undergoing Primary PCI: An Evidence-Based Clinical Approach. American Journal of Cardiovascular Drugs. 2022; 22:1-2.
- [7]Frampton J, Devries JT, Welch TD, Gersh BJ. Modern management of ST-segment elevation myocardial infarction. Current problems in cardiology. 2020; 45(3):100393.
- [8]Kchaou W, Abbès F, Attia H, Besbes S. In Vitro Antioxidant Activities of Three Selected Dates from Tunisia (Phoenix dactylifera L.). J Chem. 2014; 2014: 1–9.
- [9]Liu J, Peter K, Shi D, Zhang L, Dong G, Zhang D, et al. Anti-inflammatory effects of the chinese herbal formula sini tang in myocardial infarction rats. Evidence-based Complement Altern Med. 2014; 2014: 1–10.
- [10]Joe Y, Uddin MJ, Park J, Ryu J, Cho GJ, Park JW, Choi HS, Cha MH, Ryter SW, Chung HT. Chung Hun Wha Dam Tang attenuates atherosclerosis in apolipoprotein E-deficient mice via the NF-κB pathway. Biomedicine & Pharmacotherapy. 2019; 120:109524.
- [11]Ayanniyi R, Olumoh-Abdul HA, Ojuade FI, Asogwa N. Protective effect of Croton zambesicus leaf extract against carbon tetrachloride-induced cardiac toxicity in rats. Thai Journal of Pharmaceutical Sciences (TJPS). 2020; 44(4).
- [12]Joe Y, Zheng M, Kim HJ, Kim S, Uddin MJ, Park C, Kang SS, Ryoo S, Ryter SW, Chang KC, Chung HT. Salvianolic acid B exerts vasoprotective effects through the modulation of heme oxygenase-1 and arginase activities. Journal of Pharmacology and Experimental Therapeutics. 2012; 341(3):850-8.
- [13]Oseni OA, Ogunmoyole T, Idowu KA. Lipid profile and cardio-protective effects of aqueous extract of moringa oleifera (lam) leaf on bromate-induced cardiotoxicity on Wistar albino rats. European Journal of Advanced Research in Biological and Life Sciences Vol. 2015;3(2).
- [14]Velavan S, Selvarani S, Adhithan A. Cardioprotective effect of Trichopus zeylanicus against myocardial ischemia induced by isoproterenol in rats. Bangladesh Journal of Pharmacology. 2009; 4(2):88-91.
- [15]Van Wyk B-E. The potential of South African plants in the development of new medicinal products. South African J Bot. 2011; 77(4): 812–829.
- [16]Maikai VA, Kobo PI, Maikai BVO. Antioxidant properties of Ximenia americana. African J Biotechnol. 2010; 9(45): 7744–776.
- [17]Pendota SC, Aderogba MA, Moyo M, Mcgaw LJ, Mulaudzi RB, Staden J Van. Antimicrobial , antioxidant and cytotoxicity of isolated compounds from leaves of Pappea capensis. South African J Bot. 2017; 108: 272–277.
- [18]Pm N, Jk K, Vz S, Jk M, Dn M, Mp N. Antipyretic Potential of Methanolic Stem Bark Extracts of Harrisonia abyssinica Oliv and Landolphia buchananii ( Hallier F .) Stapf in Wistar Rats. J Appl Pharm. 2016; 8(3): 1–7.
- [19]Evans WC. Trease and Evans’ pharmacognosy. Elsevier Health Sciences; 2009.
- [20]Kirkham TC, Williams CM, Fezza F, Marzo V Di. Endocannabinoid levels in rat limbic forebrain and hypothalamus in relation to fasting, feeding and satiation: stimulation of eating by 2-arachidonoyl glycerol. Br J Pharmacol. 2002; 136(4): 550–557.
- [21]Olfert ED, Cross BM, McWilliam AA. Guide to the care and use of experimental animals. Vol. 1. Ottawa: Canadian Council on Animal Care Ottawa; 1993.
- [22]Zafar F, Jahan N, Khan A, Akram W. Cardioprotective Potential of Polyphenolic Rich Green Combination in Catecholamine Induced Myocardial Necrosis in Rabbits. Evidence-Based Complement Altern Med. 2015; 2015: 1–9.
- [23]Lazic SE, Semenova E, Williams DP. Determining organ weight toxicity with Bayesian causal models : Improving on the analysis of relative organ weights. Sci Rep. 2020; 6625(2020): 1–12.
- [24]Aebi H. Catalase in vitro. In: Methods in enzymology. Elsevier; 1984. p. 121–6.
- [25]Lowry OH. Protein measurement with the Folin phenol reagent. J biol Chem. 1951; 193: 265–275.
- [26]Wills ED. Lipid peroxide formation in microsomes. General considerations. Biochem J. 1969;113(2) :315–324.
- [27]Kang H, Shi-juan L, Jiang-hua Z, Miao W, Wei Z, Qiang L, et al. Effect of Atractylodes macrocephala extract on chronic heart failure in rats. Trop J Pharm Res. 2019; 18(10): 2075–2079.
- [28]Ayza MA, Balasubramanian R, Berhe AH. Cardioprotective Effect of Croton macrostachyus Stem Bark Extract and Solvent Fractions on Cyclophosphamide-Induced Cardiotoxicity in Rats. Evidence-Based Complement Altern Med. 2020; 2020: 1–13.
- [29]Deng J-S, Lee S-D, Kuo W-W, Fan M-J, Lin Y-M, Hu W-S, et al. Anti-apoptotic and pro-survival effect of protocatechuic acid on hypertensive hearts. Chem Biol Interact. 2014; 209: 77–84.
- [30]Nayagam AAJ, Gunasekaran S, Rangarajan S, Muthaiah S. Myocardial potency of Caesalpinia bonducella Linn. on doxorubicin induced myocardial infarction in albino rats. Clin Phytoscience. 2019; 5(1): 1–7.
- [31]Muhammad S, Shah A, Akram M, Riaz M, Munir N, Rasool G. Cardioprotective Potential of Plant-Derived Molecules: A Scientific and Medicinal Approach. Dose-Response. 2019; 17(2): 1–14.
- [32]Sutherland BA, Rahman RMA, Appleton I. Mechanisms of action of green tea catechins , with a focus on ischemia-induced neurodegeneration. J Nutr Biochem. 2006; 17(5): 291–306.
- [33]Zhang Y, Coats AJS, Zheng Z, Adamo M, Ambrosio G, Anker SD, et al. Management of heart failure patients with COVID‐19: a joint position paper of the Chinese Heart Failure Association & National Heart Failure Committee and the Heart Failure Association of the European Society of Cardiology. Eur J Heart Fail. 2020; 22(6): 941–56.
- [34]Park JW, Kim JE, Kang MJ, Choi HJ, Bae SJ, Kim SH, et al. Anti-Oxidant Activity of Gallotannin-Enriched Extract of Galla rhois Can Associate with the Protection of the Cognitive Impairment through the Regulation of BDNF Signaling Pathway and Neuronal Cell Function in the Scopolamine-Treated ICR Mice. antioxidants. 2019; 8(10): 1–20.
- [35]Krajka-Kuźniak V, Paluszczak J, Szaefer H, Baer-Dubowska W. The activation of the Nrf2/ARE pathway in HepG2 hepatoma cells by phytochemicals and subsequent modulation of phase II and antioxidant enzyme expression. J Physiol Biochem. 2015; 71(2): 227–238.
- [36]Clifford T, Acton JP, Cocksedge SP, Davies KAB, Bailey SJ. The effect of dietary phytochemicals on nuclear factor erythroid 2-related factor 2 (Nrf2) activation: A systematic review of human intervention trials. Mol Biol Rep. 2021; 48(2): 1745–1761.